Infrared astronomy has revealed much about the universe, from protoplanetary disks and nebulae to brown dwarfs, auroras, and volcanoes on multiple celestial bodies. Looking to the future, astronomers hope to conduct infrared studies of supernova remnants (SNRs) to provide important information about the physics of these explosions. Studies of near-to-mid-infrared (NIR-MIR) spectra are expected to provide data on the atomic composition of SNRs, whereas studies of mid-to-far-infrared (MIR-FIR) spectra are expected to provide information on the atomic composition of heated dust particles. You should be able to get a detailed look at the It is ejected into the interstellar medium (ISM).
Unfortunately, due to the limitations of existing infrared observatories, these studies have been mainly limited to the Milky Way and the Magellanic Clouds. However, these observation regimes are now accessible thanks to next-generation instruments such as: james webb space telescope (JWST). In a recent study, a team led by researchers at The Ohio State University published the first spatially resolved infrared images of supernova remnants (SNRs) in the Triangular Galaxy (also known as Messier 33). Their observations allowed them to obtain images with an SNR of 43, thanks to the unprecedented sensitivity and resolution of Webb’s IR instruments.
The team was led by Dr. Sumit K. Sarvadhikari, a former postdoctoral fellow in the OSU Center for Cosmology and Astrophysics (CCAP) and current research assistant at Johns Hopkins University (JHU). He has several astronomers and physicists at OSU, the Harvard-Smithsonian Center for Astrophysics, the Flatiron Institute Center for Computational Astrophysics, the Institute for Theoretical Astrophysics at Heidelberg University, the National Radio Astronomy Observatory (NRAO), and Space Telescope Science. Scholars participated. Research Institute (STScI). A paper describing their findings is under review for publication in the following journals: astrophysical journal.
![NASA/ESA/CSA's James Webb Space Telescope observed the Crab Nebula in search of answers about the origin of supernova remnants. Webb's NIRCam (near-infrared camera) and MIRI (mid-infrared instrument) have revealed new details in the infrared. Similar to Hubble's optical wavelength images released in 2005, Webb's remains show a sharp cage of fluffy red-orange gas filaments tracking doubly ionized sulfur (Sulfur III). It appears to be made up of structures. Inside the debris, fluffy yellow-white and green ridges form large loop-like structures, representing areas where dust particles reside. This area consists of a translucent milky white material. The material emits synchrotron radiation, which radiates across the entire electromagnetic spectrum but is particularly vivid thanks to the Webb's sensitivity and spatial resolution. It is produced by particles that are accelerated to extremely high speeds as they wrap around magnetic field lines. Synchrotron radiation can be tracked throughout much of the interior of the Crab Nebula. Find a wisp that follows a ripple-like pattern in the center. At the center of this ring-like structure is a bright white dot. This is a rapidly rotating neutron star. Further away from the nucleus, a thin white ribbon of radiation is traced. The curved wisps are closely grouped and follow different directions that mimic the structure of a pulsar's magnetic field. Notice that certain gas filaments are more blue in color. These regions contain singly ionized iron (Fe II). [Image description: An oval nebula with a complex structure against a black background. On the oval's exterior lie curtains of glowing red and orange fluffy material. Interior to this outer shell lie large-scale loops of mottled filaments of yellow-white and green, studded with clumps and knots. Translucent thin ribbons of smoky white lie within the remnant’s interior, brightest toward its centre.]](https://www.universetoday.com/wp-content/uploads/2023/10/Crab-1-1024x892.jpeg)
As they explain in their study, the SNR of the Milky Way and the Magellanic Clouds are the most well-studied because they are the closest in the universe. This has allowed astronomers to conduct detailed studies that reveal structure at most wavelengths, including infrared. As Dr. Sarvadhikary told Universe Today via email, studying these SNRs has taught astronomers a lot. This includes the physics of dust formation, the composition of supernova explosions, and especially the astrophysical shock waves that travel through dense gas clouds where new stars can form.
However, as Sarvadhikari explained, these studies are still limited to the Milky Way and its moons, which limits what astronomers can learn about these major astronomical phenomena.
“[The] The only problem is that we haven’t yet been able to go outside the Magellanic Clouds and explore the SNR of more distant galaxies in the infrared. Other local group galaxies such as Andromeda (M31) and Triangulum (M33) are known to have SNRs in the hundreds, so the potential for building statistics is very high. Additionally, infrared-emitting SNRs are a somewhat rare type, primarily due to either explosions occurring near dense molecular gas that is part of the interstellar medium, or material lost by the progenitor star before the explosion. It happens. Therefore, having more objects becomes very useful. ”
The first generation of SNR studies at infrared wavelengths were conducted using NASA’s Infrared Astronomy Satellite (IRAS) and ESA’s Infrared Space Observatory (ISO). Despite limited spatial resolution and turbulence peering into the galactic plane, these observatories were able to identify about 30% of the Milky Way’s SNR between 10 and 100 micrometers (?m). This corresponds to part of the mid-infrared and far-infrared rays. (MIR, NIR) spectrum.

In recent decades, IR astronomy has benefited greatly from missions like NASA’s. Spitzer Space Telescope and ESA’s Herschel Space Observatory. These observatories boast higher angular resolution and can survey a wider part of the IR spectrum, from 3 to 160 μm for Spitzer and from 70 to 500 μm for Herschel. Their observations include the Galactic Heritage Infrared Midplane Survey Extraordinaire (GLIMPSE), the MIPS Galactic Surface Survey (MIPSGAL), and the Herschel Infrared Galactic Surface Survey (Hi-GAL), as well as the first high-quality extragalactic survey. It led to IR study of SNR.
“Unfortunately, the angular resolution of the Spitzer Telescope (JWST’s predecessor) was not sufficient to recover the same spatial details in more distant galaxies,” Sarvadhikary added. “You might see a faint blip with Spitzer, but it would be difficult (at this distance) to tell whether it’s due to SNR or a mix of stellar and diffuse radiation.” Fortunately, , this situation is james webb space telescope (JWST). Sarbadhicary said Webb’s increased resolution and advanced IR instrumentation will provide a deeper and clearer view of SNR at near-infrared and mid-infrared wavelengths.
“We have already recognized the potential of JWST to revolutionize SNR research from the sharp new images of known SNRs, such as Cassiopeia A in the Milky Way and 1987A in the Large Magellanic Cloud, published in recent papers. These images revealed an unprecedented amount of detail about the explosion debris, the material the star lost before it exploded, and more.
“This superior combination of sensitivity and angular resolution allows JWST to image the SNR of galaxies nearly 20 times more distant than the Magellanic Clouds (for example, M33 in the paper) with the same level of detail that Spitzer found for the Magellanic SNR. Now you can restore it. cloud. Particularly useful due to JWST’s high angular resolution is that it is less likely to confuse SNR with overlapping structures such as HII regions (gas photoionized by massive stars). ”
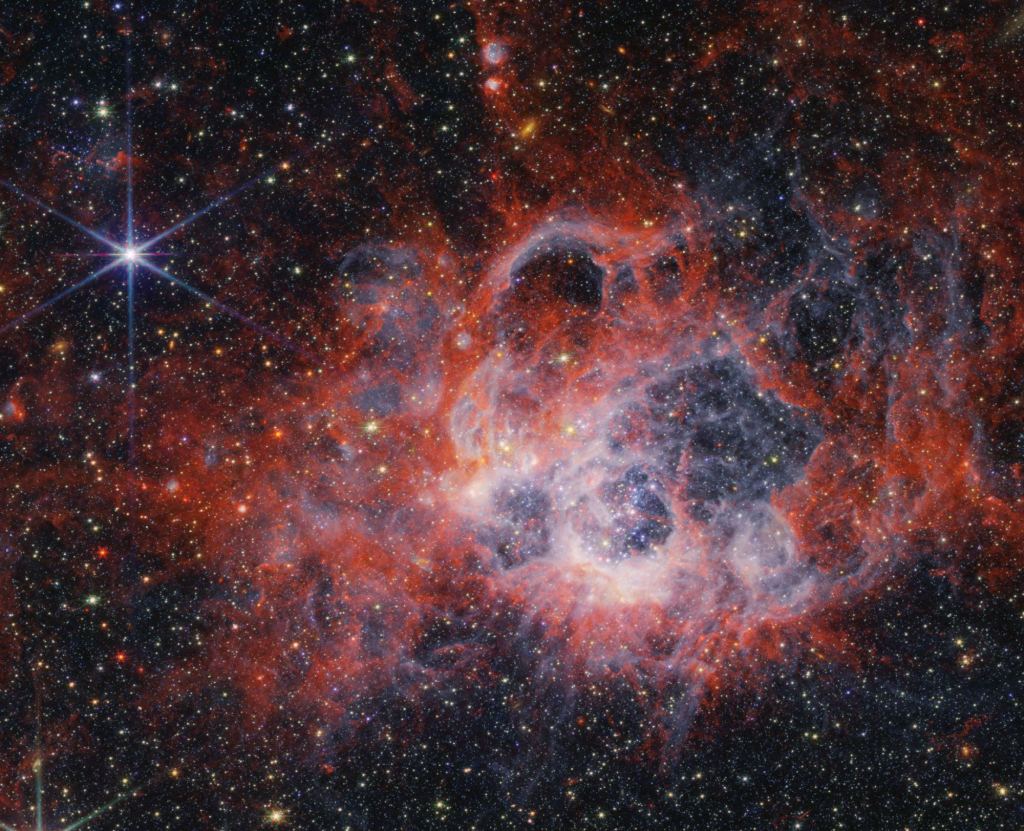
For their study, Sarvadhikari and his team leveraged archived JWST observations of the Trangulum galaxy (M33) in four JWST fields. Two of the observations were separate observations using Webb’s Near-Infrared Camera (NIRCam) and its Mid-Infrared Imager (MIRI), covering the central and southern regions of M33. The third was a long radial band of MIRI observations, about 5 kiloparsecs (about 16,300 light-years), one of which covered the giant emission nebula of M33 (NGC 604) with multiple NIRCam and MIRI observations. We then overlaid these observations with previously identified SNRs from multiwavelength surveys.
They also considered the amount of multiwavelength data obtained from this galaxy in previous missions. This also includes images of stars obtained by the Venerable. hubble Finally, there are cold neutral gas observations performed by the Atacama Large Millimeter-submillimeter Array (ALMA) and the Very Large Array (VLA). As Sarbadhicary pointed out, the results revealed some very interesting things about the SNR of triangular galaxies. However, they also noted that these results are just the tip of the iceberg, as the study only covered 20% of M33’s SNR.
“The most surprising finding was that in two of the three SNRs observed with F470N (a narrowband filter centered around the 4.7-micron rotation line of the hydrogen molecule), molecular hydrogen emissions were present. Although it is the most abundant molecule in interstellar gas, H2 cannot produce visible light at the typical low temperatures of interstellar gas, but only when heated by bombardment or ultraviolet radiation. (e.g., 4.7 microns), making it extremely useful for tracking the impact of bombardments on dense molecular gas where star formation occurs.”
Astronomers have observed this emission at several SNRs in the Milky Way, but this is the first time such an observation has been made at an extragalactic source. “JWST data also revealed that 14-43% of the SNR exhibits visible infrared radiation,” Sarbadhicary added. “The brightest infrared SNRs in our sample are some of the smallest in M33, and the brightest at other wavelengths, especially X-ray, radio, and optical. This is because the impact of these SNRs is still relatively small. This means that they travel at high speeds and collide with dense material in the environment, radiating significant amounts of impact energy into infrared radiation and into the dust, illuminating the radiation seen in broadband images.”

The results demonstrate how the high angular resolution of the Webb allows astronomers to perform high-precision infrared observations of large numbers of SNRs in galaxies beyond the Magellanic Clouds. This includes M33, the Andromeda Galaxy (M31), and nearby Local Group galaxies such as the Southern Pinwheel Galaxy (M83), the Fireworks Galaxy (NGC 6946), the Spiral Galaxy (M51), several dwarf galaxies within the Local Group, and others. Contains many galaxies. more! Sarvadhikari said:
“Personally, the physics of how shocks affect dense gas and control star formation in galaxies is a major topic in astronomy, so I used JWST to study how shocks affect dense gas and control star formation in galaxies. We are very excited to be able to study a population of SNRs that give ions at infrared wavelengths, a treasure trove of ion and molecular beams (such as the H2 we discovered) that are excited by shocks in warm, dense gas clouds. These observations are very useful.
“These galaxies also have some rare Cassiopeia A-like SNRs that are very young and rich in ejecta from explosions, and JWST can provide a lot of new information from the infrared emission lines. Another A big area of research is dust and how it is created and destroyed by impact.”
More information: arXiv